This is the multi-page printable view of this section. Click here to print.
Physics
- 1: Electrostaitcs
- 1.1: Electric charge
- 1.2: Coloumb's Law
- 2: Statitical Mechanics notes
- 3: Nuclear Physics CSIR-NET prep notes
- 3.1: Nuclear Radius
- 3.2: Nuclear Spin and Parity
1 - Electrostaitcs
1.1 - Electric charge
What is electric charge?
We have three types of particles in nature on the basis of electrostatics. They are namely positive charge particles, negatively charged particles, and neutral particles. They are determined in such a way based on their interaction i.e.
-
like charges repel each other,
-
Unlike charges attract each other, and
-
neutral bodies don’t interact with any other particles.
I.e. Two positive charges always repel each other, and so do the negative charges. Whereas a positive charge always attracts negative charge and vice versa.
Neutral particles are combinations of both positive and negative charges in equal amounts or mathematically we call 0 charge or no charge. As positive attracts negative and negative attracts positive zero being neutral it does not interact with any charge. For example a neutron will not interact with either an electron or a proton.
Informal definition: From the above information we can understand that charge is a physical quantity of particles which determines the nature of electrical interactions.
SI unit of charge is coulomb and represented as C. An electron has a charge of $-1.6 \times 10^{-19}$ C and a proton has a charge of $+1.6 \times 10^{-19}$ C. Whereas a neutron charge is 0 C
*Note: positive and negative is just a representation of the opposite nature of particles in a mathematical way.
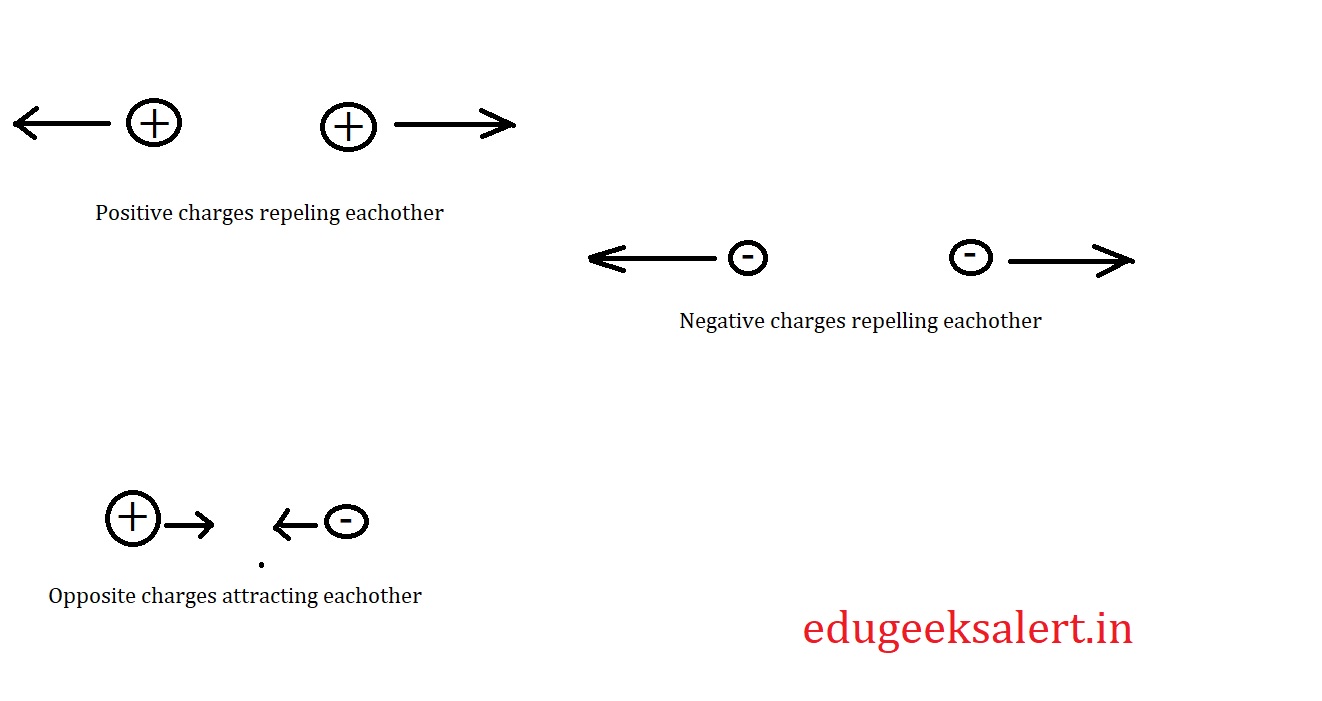
Quantization of charge:
Every particle in the universe is made up of atoms, which are made up of electrons, protons and neutrons. Electron is the smallest negative charge while proton is the smallest positive charge there for any charge should be integral multiple of these particles that is a negatively charged particle should be -n e where and is a positive integer and $e = 1.6 \times 10^{-19}$ C. similarly positive charge should be +n e where and is positive integer and $e = 1.6 \times 10^{-19}$. This means that the fractional charge of e doesn’t exist.
Charge is conserved:
A proton and electron can come by to form a Neutron. Similarly a neutron can be divided into a proton and an electron. And in atoms, protons and neutrons makeup the nucleus while electrons revolve around the nucleus in orbits to form a neutral atom.
By this we can understand that charges can be neutralized and charge can be formed from neutral bodies. But the net charge of a closed system is always the same. i.e. net charge of a closed system can neither be destroyed nor created.
Additivity of electric charge:
When two charges combine together the charge of combination is the algebraic sum of the charges of two bodies. i.e. when a 5 C charge body meets with 6 C charge body and stays together then the resultant charge of the new body is 11 coulombs. Similarly when a 5 C charge body meets with -6 C charge body and stays together then the resultant charge of the new body is -1 coulombs.
A real life example of all the above properties of charges can be observed in beta decay. When a neutron in the nucleus of a heavy atom splits into a proton and an electron the charge is conserved because the charge before splitting is zero and the charge after splitting as a whole is 0. Is an example of conservation of charge and an example of additivity of charge.
Conduction of charge:
When a charged body is made contact with a neutral conducting body, Charge flows to the neutral body so that charge density is equally distributed in the both bodies. this can be understood by following image.
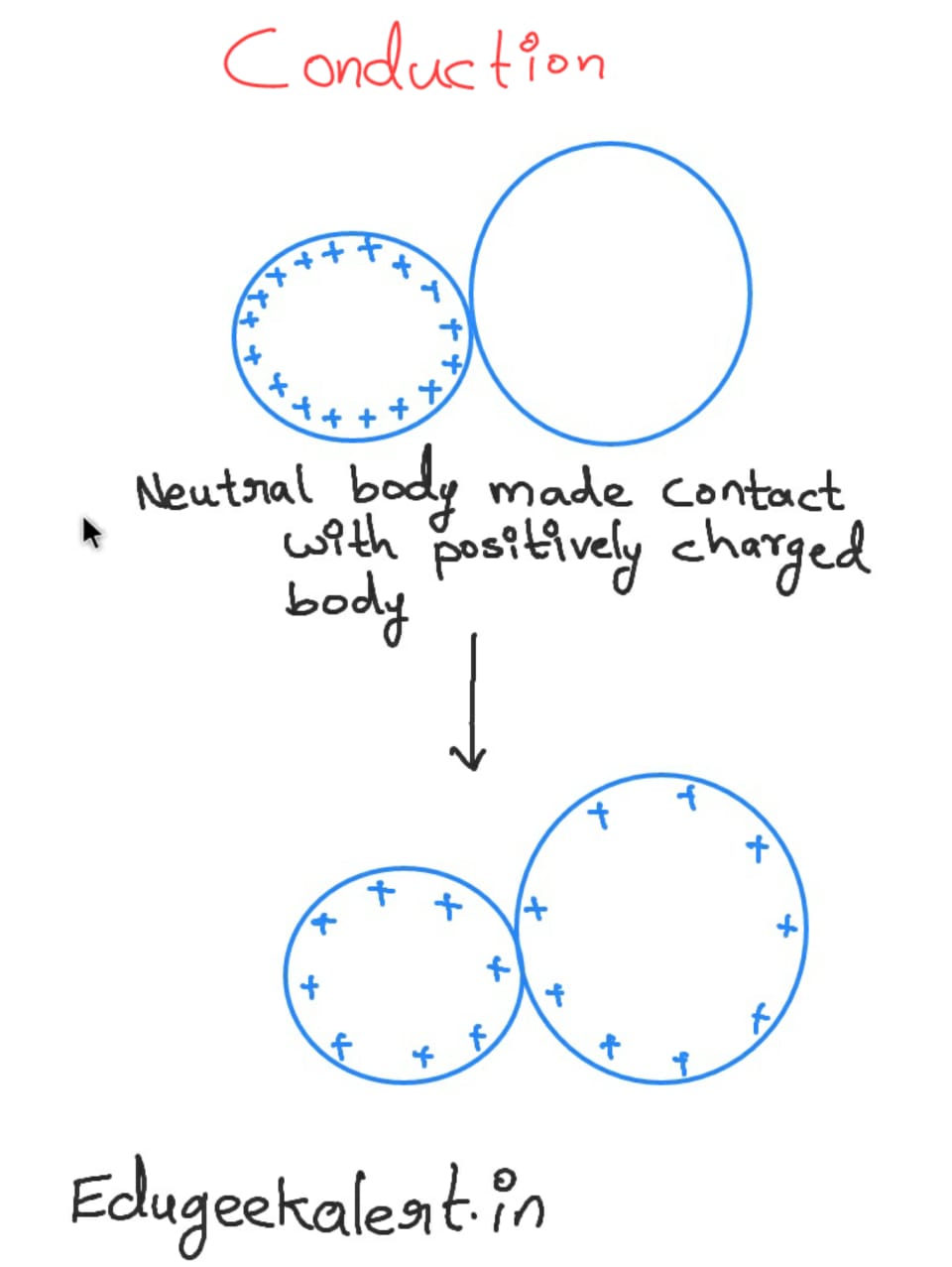
Induction of charge into a neutral body:
Charge can be induced into a body not only by conduction. when a neutral body is brought into the filed of a positively charged body, as shown in the figure, electronss inside the neutral body get attracted towards the positive charge and postive charge get repeled to the otherside as shown in the figure. when we ground the negative charge end, the electrons would floiw to the ground making the body postively charged.
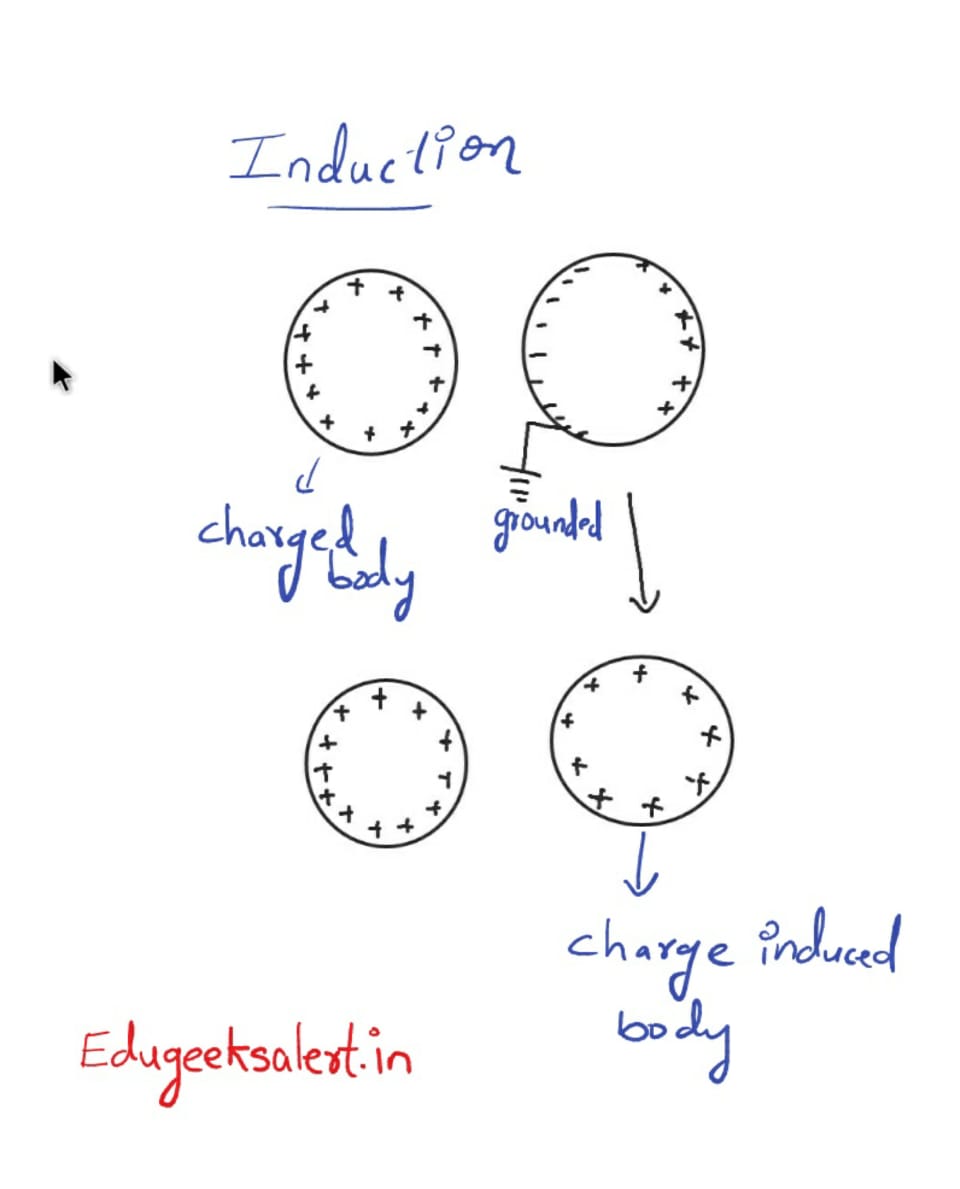
1.2 - Coloumb's Law
Coulomb’s law:
The results of many experiments done by Coulomb and many other scientists developed a relation of force exerted by a charged particle on another charge. It is given as:
$$F \propto \frac{q_1 q_2}{r^2}$$
Where $q_1$ and $q_2$ charges of the particles and r is the separation between them. We can write the above proportionality as follows:
$$F = \frac{k q_1 q_2}{r^2}$$
Where k is the proportionality constant. The vectorical representation of coulomb’s law can be written as :
$$\vec{F} = \frac{kq_1 q_2}{r^3} \vec{r}$$
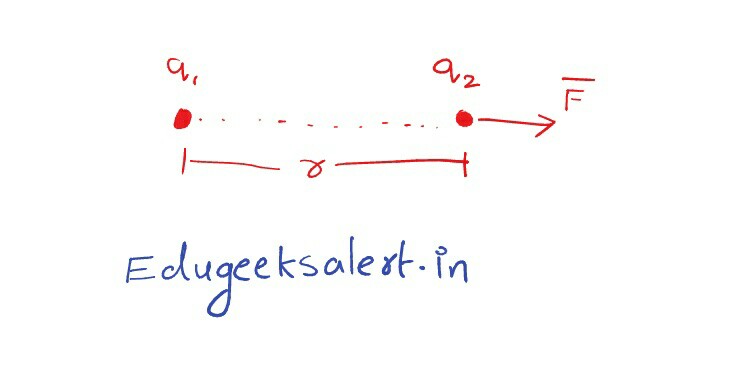
When the constant value was measured experimentally it was found to be $\frac{1}{4\pi \epsilon_0}$. Where $\epsilon_0$ is the permittivity of free space, $\epsilon_0=8.85419\times 10^{-11} C^2 N{-1} m{-2}$. By substituting the constant we get:
$$F = \frac{1}{4\pi \epsilon_0} \frac{ q_1 q_2}{r^2}$$
and vectorically,
$$\vec{F} = \frac{1}{4\pi \epsilon_0} \frac{ q_1 q_2}{r^3} \vec{r}$$
Practice problems
-
A Charge A of 5 C is placed at origin and another charge B of 5 C is placed at (5,0) on the cordinate plane. Find the electrical force acting on A and it’s direction.
-
find the force acting on B and its direction in the above question
-
Three charges of charge q are placed on the vertices of an equilateral triangle of edge a. Find the force acting on one charge because of other two charges.
-
three charges of charge q are placed on three vertices of square of side length a then find the force acting on test charge Q placed at the centre of the square.
-
by what distance two charges of charge 1C each must be separated so that force between them is equals to 50 N.
-
Two charges, 5 C and 6 C are separated by distance 1 m. At what point the third charge can be placed so that it experiences no net force.
2 - Statitical Mechanics notes
2.1 - Prerequisites and Historical note
This is the notes is the made using lectures of various premium institutes across India.
Prerequisites
- Various mathematical concepts such as Advanced calculus which includes multivariable integrations, complex variables, combinatronics and so on.
- Basic programming in a language.
- Knowledge of Classical Mechanics and, Quantum Mechanics upto undergraduate level. ( you can refer to Classical Mechanics by Herbert Goldstein and Introduction to quantum mechanics by David J. Griffiths)
Historical Note:
- Historically, Thermodynamics was the conceptual preceder to Statistical Mechanics.
- Since the antiquity to 1600 A.D. notion of heat and temperature were widely accepted. It was believed at that time that heat was associated with the motion of microscopic constituents of matter
- Later during 1700s and first half of 1800s,the wrong notion that heat was instead a fluid like substance started becoming popular.
- In 1850 experiments of James Joule and others show that heat is a form of energy.
- Sadi Carnot had explained the relation between heat and energy. Which was important in the development of steam engines.
- In 1850 Rudolf Clausius and William Thomson (Lord Kelvin) formulated the first law which is the idea that total energy is conserved. The second law of thermodynamics which is the idea that heat cannot be completely converted to work was also formulated.
- In 1738 Daniel Bernoulli has pointed out that gases consist of molecules in motion. Clausius revived this idea in 1857.
- In 1860 James clerk Maxwell derived the expected distribution of molecular speeds in a gas by taking into account molecular conditions.
- In 1877 Ludwig Boltzmann constructed an equation that he thought could describe the detail time evolution of a gas regardless of whether it was in equilibrium or not.
- In the 1860, Clausius had introduced entropy as a ratio of heat to temperature, and had stated the second law in terms of the increase of this quantity.
- Boltzmann then showed that his equation implied the so called H theorem, which states that a quantity equal to entropy in equilibrium must always increase with time. Since molecular collisions were assumed reversible, his derivation could be run in reverse, and would then imply opposite of second law. Boltzmann made the implicit assumption that the motion was uncorrelated before collision but not after which imposes irreversibility.
- In 1900, Gibbs introduced the notion of an ensemble - a collection of many possible states of a system, each assigned a certain probability.
He argued that if the time evolution of a single state were to visit all other states in the ensemble, the so called ergodic hypothesis - then
averaged over a sufficiency long time a single state would behave in a way that was typical of the ensemble. Gibbs also gave qualitative arguments that entropy would increase if it were measured in a “coarse grained” way in which nearby states were not distributed.
3 - Nuclear Physics CSIR-NET prep notes
3.1 - Nuclear Radius
As we nucleaus is the core of the atom, where most of the mass of the atom is concentrated, and the nuclear radius is an essential concept in nuclear physics, as it represents the size of the atomic nucleus. It plays a significant role in understanding various nuclear phenomena, such as nuclear reactions, nuclear decay, and nuclear fission. This essay aims to provide a detailed explanation of the nuclear radius, its measurement, and its significance in nuclear physics.
The nuclear radius is the distance between the center of the nucleus and its outermost boundary, or the point at which the nuclear density decreases to a negligible value. The nuclear radius is much smaller than the atomic radius, which is the distance between the nucleus and the outermost electron in the atom. The atomic radius is typically on the order of angstroms (10^-10 meters), while the nuclear radius is on the order of femtometers (10^-15 meters).
Nuclear radius increases with the increase in the nucleons, i.e. atom with higher nucleons has higher radius than those of the lesser ones. However, this increase is not linear but follows a more complex trend due to the strong nuclear force that holds the nucleus together. The strong nuclear force is a short-range force that binds the nucleons together and is much stronger than the electromagnetic force that repels the positively charged protons from each other. As a result, the nuclear radius increases less rapidly than the number of nucleons, and the nuclear density decreases as the nucleus becomes larger.
Measurement of Nuclear Radius:
The measurement of nuclear radius is a challenging task due to the small size of the nucleus and the difficulty in probing its structure. Several methods have been developed to determine the nuclear radius experimentally, each with its advantages and limitations.
One of the most common methods for measuring nuclear radii is through electron scattering experiments. In this technique, high-energy electrons are directed at a thin foil of the element under investigation, and the scattered electrons are detected at different angles. The distribution of scattered electrons provides information about the size and shape of the nucleus. The advantage of this method is that it can be used for a wide range of nuclei and can provide information about the nuclear charge distribution. However, the electron scattering method is limited by the resolution of the detectors and the complexity of the nuclear structure.
Further information:
Another method for measuring nuclear radii is through the analysis of nuclear decay processes. In radioactive nuclei, the decay process involves the emission of particles from the nucleus, such as alpha or beta particles. The energy and momentum of these particles depend on the size and structure of the nucleus. By analyzing the decay products, one can determine the nuclear radius indirectly. The advantage of this method is that it can provide information about the excited states of the nucleus and the distribution of nuclear spins. However, the interpretation of the data can be complex, and the method is limited to radioactive nuclei.
A third method for measuring nuclear radii is through the study of nuclear collisions. In this technique, two nuclei are accelerated to high energies and made to collide with each other. The trajectory and energy of the scattered particles can provide information about the nuclear radius and the strength of the nuclear force. The advantage of this method is that it can provide information about the collision dynamics and the nuclear matter distribution. However, the interpretation of the data can be complex, and the method is limited to a small range of nuclei.
3.2 - Nuclear Spin and Parity
Spin is a quantum mechanical property that describes the intrinsic angular momentum of a nucleus, while parity is a quantum number that describes the symmetry properties of a nucleus under reflection. The spin and parity of a nucleus play a crucial role in determining its physical and chemical properties and are used extensively in nuclear physics research.
Spin:
The spin of a nucleus is a fundamental property that arises from the intrinsic angular momentum of its constituent particles, such as protons and neutrons. The spin of a nucleus is described using the quantum mechanical concept of angular momentum, which is a vector quantity that represents the rotation of an object around an axis. The magnitude of the angular momentum of a nucleus is proportional to its spin quantum number, denoted by the symbol I.
The spin quantum number can take on half-integer or integer values, depending on the number of protons and neutrons in the nucleus. For example, nuclei with an odd number of protons or neutrons have a half-integer spin, while nuclei with an even number of protons and neutrons have an integer spin. The spin quantum number is important in nuclear physics because it determines the magnetic moment of the nucleus, which is used in various experimental techniques, such as nuclear magnetic resonance (NMR) spectroscopy.
Parity:
The parity of a nucleus is a quantum number that describes its symmetry properties under reflection. A nucleus can be either even or odd parity, depending on whether it is symmetric or asymmetric under reflection. Parity is described using the quantum mechanical concept of parity operator, denoted by the symbol P, which changes the sign of the wave function of a nucleus under reflection.
The parity of a nucleus is related to the number of nucleons with spin up and spin down. For example, if a nucleus has an even number of nucleons with spin up and an even number of nucleons with spin down, it has an even parity. Conversely, if a nucleus has an odd number of nucleons with spin up and an even number of nucleons with spin down, it has an odd parity.
let $\psi$ (x,y,z) be a wave function representing the particle
then if $\psi$ (-x,-y,-z) = $\psi$ (x,y,z) : even parity
and if $\psi$ (-x,-y,-z) = - $\psi$ (x,y,z) : odd parity